Action Potentials
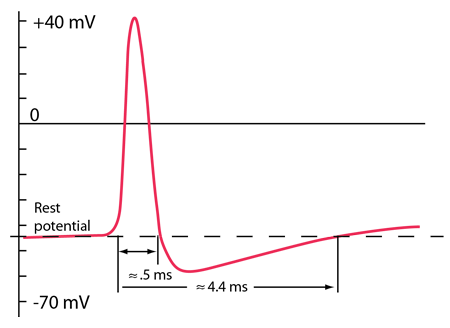 |
Action potential from a giant squid axon.
| |
In response to the appropriate stimulus, the cell membrane of a nerve cell goes through a sequence of depolarization from its rest state followed by repolarization to that rest state. In the sequence, it actually reverses its normal polarity for a brief period before reestablishing the rest potential.
The above example of the squid action potential was patterned after a measured action potential shown in West's Medical Physics. The approximate time intervals shown were scaled from time markers on the experimental trace.
The action potential sequence is essential for neural communication. The simplest action in response to thought requires many such action potentials for its communication and performance. For modeling the action potential for a human nerve cell, a nominal rest potential of -70 mV will be used. The process involves several steps:
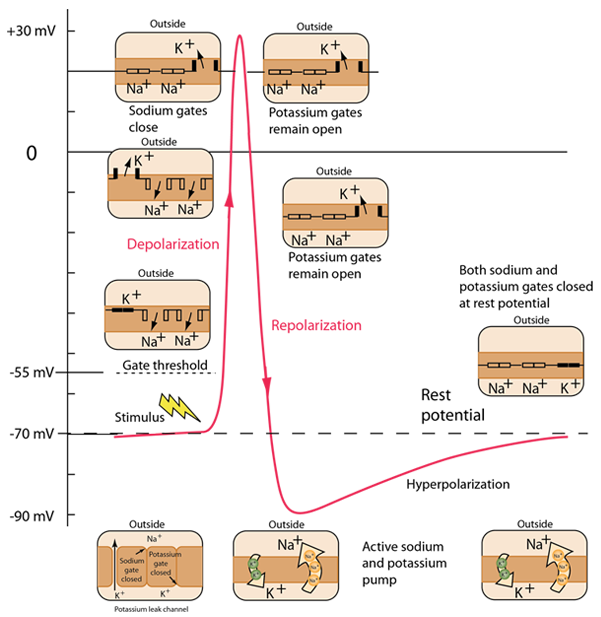 This is an active graphic. Click for more detail.
The following discussion is an attempt to characterize the successive steps involved in the production of an action potential typical of mammalian nerve cells.
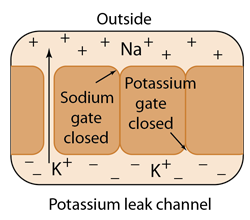 | In the resting state of a nerve cell membrane, both the sodium and potassium gates are closed and equilibrium concentrations are maintained across the membrane. Inside the cell, the K+ concentration is higher, nominally 100 mM compared to 5mM outside the cell. Outside the cell, the Na+ concentration is higher, nominally 150 mM compared to 10 mM inside the cell. The voltage or electric potential of the inside of the cell compared to the outside is typically about -70mV, although this differs significantly in cells other than nerve cells. Although the changes in electric potential across the membrane during an action potential are sufficient to accomplish its purpose, the actual changes in the concentrations of the Na+ and K+ ions are very small. They are like tiny ripples on the concentration seas. Karp, Section 4.8 describes the fact that there are some remaining open K+ channels even in the resting membrane, and they make a contribution to determining the resting potential. They are often called the K+ leak channels. |
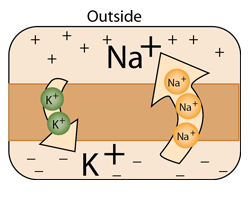 | The Na+/K+ pump is a major contributor to the resting state or equilibrium of the cell. It is responsible for maintaining the large excess of Na+ outside the cell and the large excess of K+ ions on the inside. It is an active transport process which makes use of the ATP energy currency of the cell. |
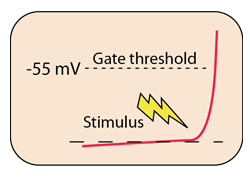 | A stimulus is received by the dendrites of a nerve cell. This causes some Na+ channels to open, and the diffusion influx of Na+ ions starts to drive the potential of the interior more positive. The nerve cells are sensitive to external stimuli, but if such stimuli do not cause the potential to rise to the threshold level, the cell will tend to settle back down to equilibrium. If the stimulus response is sufficient to drive the interior potential from -70 mV up to -55 mV, the process continues. Having reached the threshold, there is no turning back and the cell will fire into the full action potential. This situation is often referred to as the "all-or-none" law. |
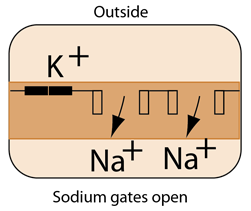 | Once the threshold is reached, more sodium gates open and Na+ ions flood into the cell, raising the voltage rapidly. This transition is said to be "voltage gated" and occurs rapidly since the Na+ ions are driven by both the concentration gradient and the voltage gradient. |
Depolarization | The period of rapid rise of the interior cell potential from its resting state of about -70 mv on the order of milliseconds is referred to as "depolarization". The rise may be to about +30 mV. The typical time for the depolarization of a mammalian nerve cell is on the order of a millisecond. |
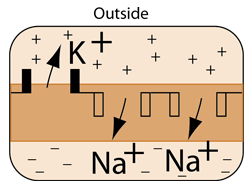 |
The open sodium gates continue to transport Na+ ions into the cell, driving the depolarization and raising the potential of the cell's interior. At some point the K+ gates begin to open, but their opening process is slower than that of the Na+ gates, so the depolarization process has time to come to completion. The depolarization continues until the interior cell potential goes positive to about 30mV. |
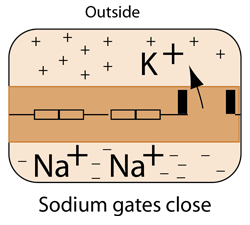 | As the interior cell potential moves through neutrality to positive values, the sodium gates close, ending the depolarization phase. |
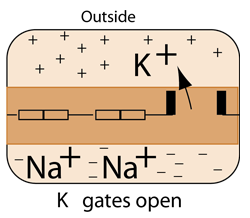 | Once open, the K+ gates remain open and the free diffusion of potassium out of the cell drives the potential back toward negative values in the process called repolarization. |
Repolarization | The drop in the interior cell potential as a result of the open K+ gates is called repolarization. This re-establishes the "polarization" of having the interior of the cell polarized negative with respect to the outside. |
Hyperpolarization | Hyperpolarization is the name given to the period of overshoot of the interior cell potential to values more negative than the normal rest state. One influence in this phase is the fact that the Na+ gates remain closed and the lack of Na+ mobility across the membrane causes the K+ process to proceed toward the value of -80mV which would occur if potassium alone were present. While hyperpolarization might seem to be counterproductive, it is actually important in the transmission of information. Hyperpolarization prevents the neuron from receiving another stimulus during this time, or at least raises the threshold for any new stimulus. Part of the importance of hyperpolarization is in preventing any stimulus already sent up an axon from triggering another action potential in the opposite direction. In other words, hyperpolarization assures that the signal is proceeding in one direction. |
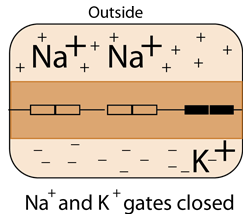 | In the hyperpolarization stage, the K+ gates close and the Na+ gates remain closed. Under the influences that control the equilibrium potential (the Na+/K+ pump and the K leakage channel), the cell membrane again approaches its rest state. |
Having completed the action potential sequence, the Na+ gates remain closed for a time, suppressing the initiation of a new action potential for a period referred to as a "refractory period".
Contributing author: Ka Xiong Charand
|
Index
Bioelectricty
Karp, 4.7-4.8 |